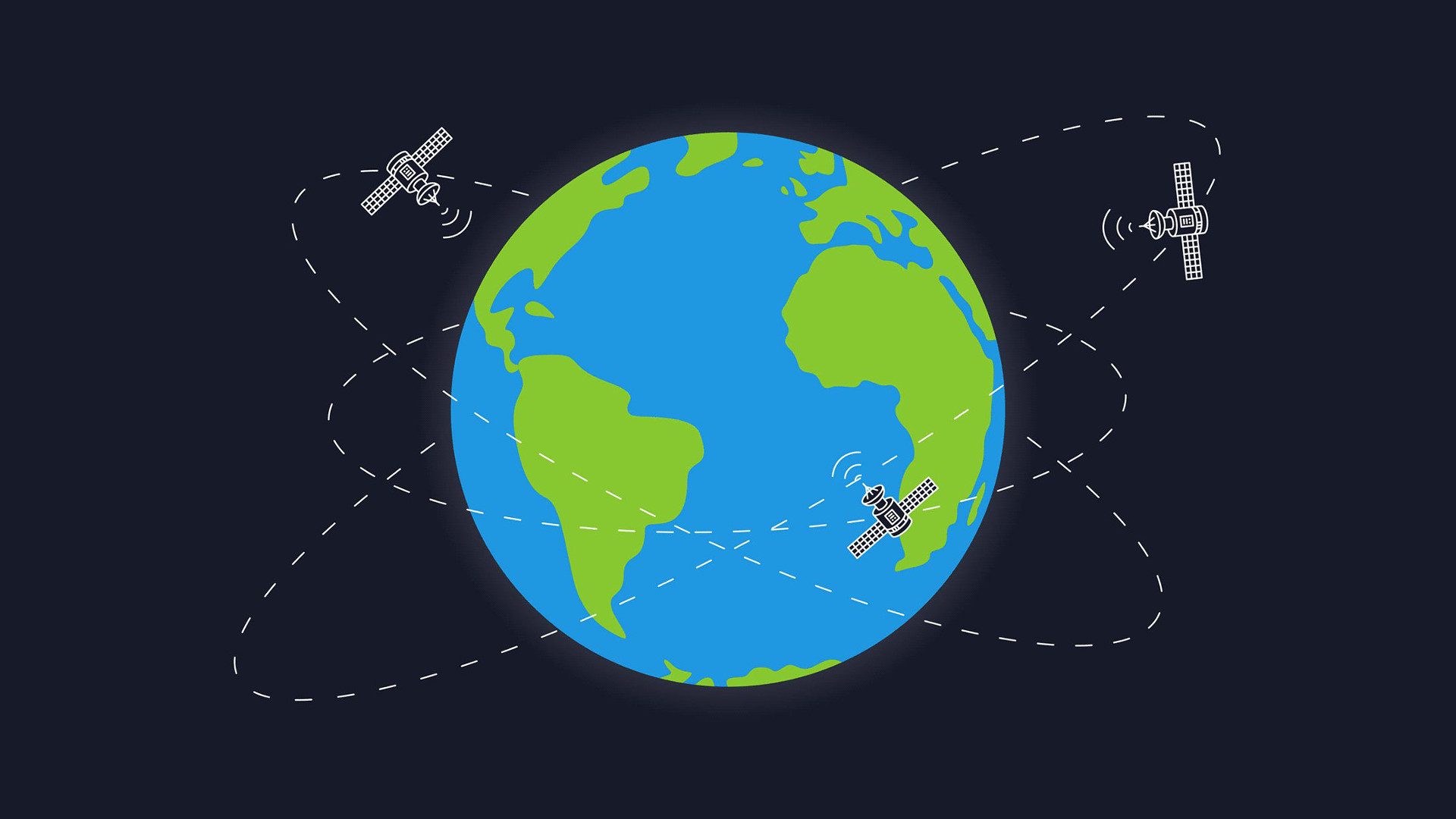
How does GPS work?
Contents: How does GPS work?
1. GPS or GNSS? *
2. GPS – an overview *
3. Where are the satellites?
4. Where is the GPS receiver?
5. Satellite geometry
6. What are we waiting for?
7. Hot and cold starts
8. What’s the time?
9. It’s all relative
* New to GPS? At least read 1 and 2.
GPS or GNSS?
We often say ‘GPS’ when talking about satellite navigation systems. However, the ‘Global Positioning System’ is actually the name of the first satellite-based navigation system. For a while, the American GPS system, which uses a satellite constellation called NAVSTAR, was the only one in existence.
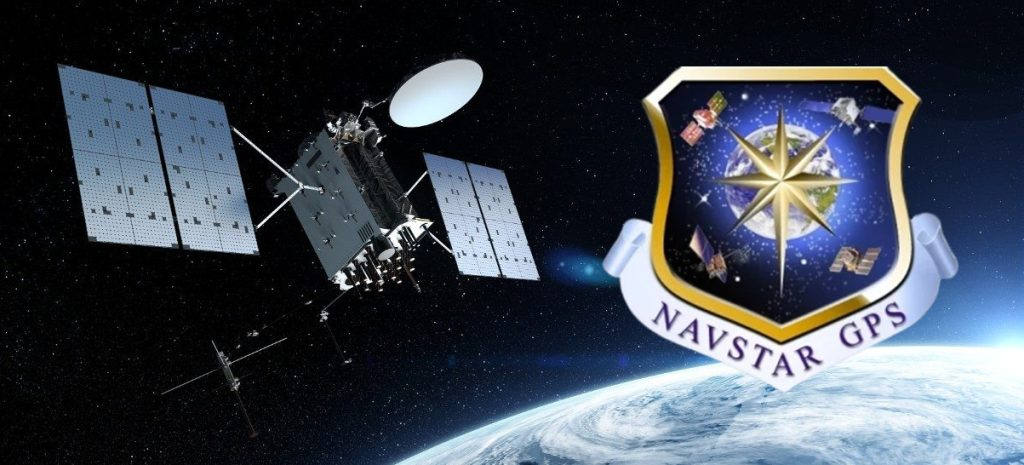
By Sept 2020 there were three other similar systems offering global coverage. GLONASS is the Russian system. BeiDou is the Chinese system and Galileo is the name of the EU’s system. India’s IRNSS/NavIC and Japan’s QZSS systems should offer global coverage in the future but currently (Jun 2023) they only provide services to those more local regions.
A modern ‘GPS’ receiver will typically use satellites from at least two of the global systems because using more satellites makes accuracy more reliable and getting a location fix faster.
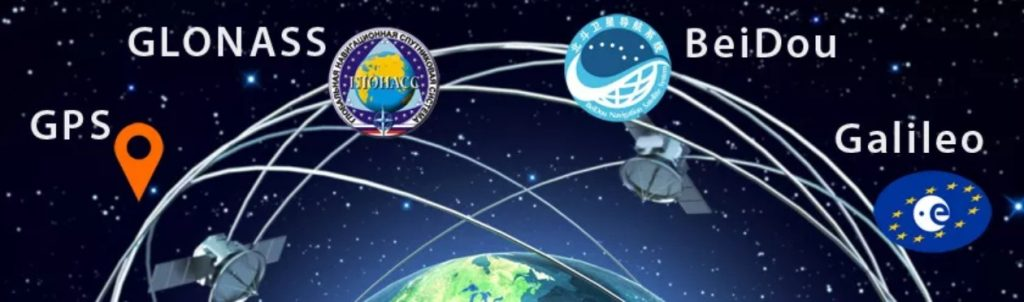
The phrase describing a satellite system that offers navigation across the whole planet is ‘Global Navigation Satellite System’ or GNSS. However, when was the last time you heard someone refer to the ‘GNSS’ on their phone or in their car? ‘GPS’ is commonly used when talking about GNSSs like Hoover is used when discussing vacuum cleaners.
I’ve probably used GPS on this website through habit when I’m not necessarily talking specifically about the American GPS system but I’ll use GNSS if it’s confusing not to.
GPS – an overview
Now I am talking specifically about the American system. Understanding the basics of America’s GPS is enough to know how to get the best from your phone or GNSS handheld as all global navigation satellite systems work in a similar way.
There are three parts to the GPS system.
- The users. You and I on the ground, armed with our satnav receivers. They could be smartphones or tablets, dedicated GNSS handhelds or GNSS watches.
- A satellite constellation. Each satellite continuously transmits the time of day and satellite location data to earth.
- A worldwide network of ground-based facilities which monitor and control the satellites.
At it’s heart is the constantly moving constellation of at least 24 operational GPS satellites. 24 is the minimum number to assure global coverage but according to the U.S. government’s GPS.gov “As of April 12, 2022, there were a total of 30 operational satellites in the GPS constellation”.
Each satellite orbits the earth twice a day travelling at 14,000 km/hr (8700 mph) at an altitude of approximately 20,200 km (12,550 miles).
The shifting constellation ensures there will always be at least four satellites visible from any point on earth, usually more. Each satellite uses radio waves to continuously broadcast the time of day, as well as information about exactly where it is and roughly where all the other satellites are too.
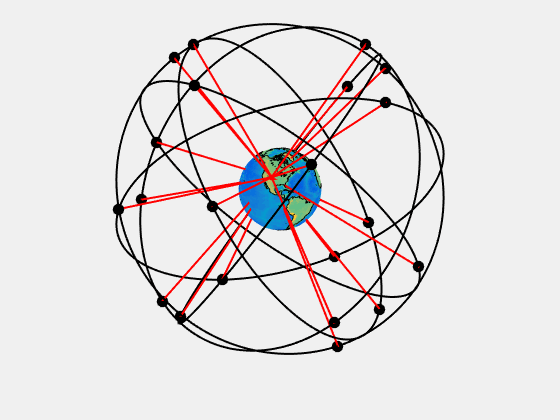
Our consumer GNSS receivers pick up these signals with little built-in aerials, just like TVs and radios pick up radio signals. The aerial feeds a tiny receiver chip. This electronic chip converts the signal to the GPS data, crunches the numbers and then provides an accurate GPS location for the device to use.
Smartphone, handheld or GNSS watch, all work the same way. Note that which app you use on a smartphone is irrelevant to positional accuracy. It’s the hardware on the phone which works out where you are. A phone app just shows that position on a map.
The GNSS receiver in your phone or handheld doesn’t ‘communicate’ with the satellites. A phone doesn’t transmit anything. GNSS receivers just ‘listen’. No cellphone signal or internet connection is required.
On earth, a received signal from a particular satellite tells your GNSS device precisely what time the signal left the satellite and exactly where the satellite was when it sent it. The difference between the time the signal left the satellite and it’s time of arrival at the receiver is how long the signal has taken to travel to earth. Multiplying that travel time by the speed of light, which is how fast radio waves travel, tells the receiver it’s distance from the satellite.
Knowing the distance to just three satellites allows the GNSS receiver to calculate an approximate position on earth (a 2D fix). Once a receiver ‘acquires’ a fourth satellite, it can make a more accurate calculation that includes elevation as well (a 3D fix).
Four satellites are the minimum for an accurate ‘3D’ fix.
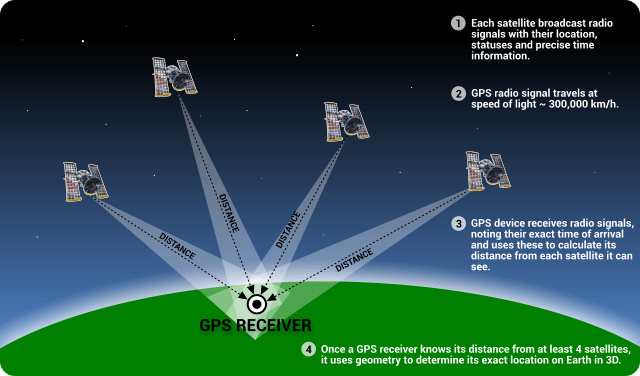
Big TV transmitters might use 200,000 watts of power to beam signals to our TVs. GPS satellites are solar powered and transmit with about the same power as a domestic light bulb. About 50 watts. Consequently the satellite signals arriving on earth are very weak. They can be easily blocked by buildings, cliffs or dense tree cover.
You must be outside with a clear view of the sky for GPS to work properly.
The three other global navigation satellite systems have different satellite arrangements but work in a similar way.
All offer great accuracy. Under an open sky, an old Garmin handheld using just the American system will typically provide a position that’s within 15 metres of where you actually are. A modern smartphone or dedicated GNSS unit will usually be less than 5 metres out. Amazing.
That’s enough to understand how to get the best from your GNSS devices but if you’d like more detail then read on…
Where are the satellites?
As well as broadcasting the time, each satellite identifies itself and transmits up-to-date information that describes its orbit. Exactly where it is at any time.
Think of that location data like a train timetable. If a friend is visiting you on the train and they tell you which train they’re on, then if you know the time of day, consulting the train timetable gives you the friend’s location.
Satellite orbits are reliably more predictable than trains. When a GPS receiver gets a time signal from a known satellite it can consult its stored ‘timetable’ for that satellite and learn exactly where the satellite was at the time the signal was sent.
Your GNSS receiver uses the time signals and stored timetable to locate itself, so this data must be very accurate. It is updated on the satellites about every 2 hours and an up -to-date satellite ‘timetable’ remains valid on your device for about 24 hours. This accurate ‘timetable’ for each satellite is called ‘ephemeris’ data.
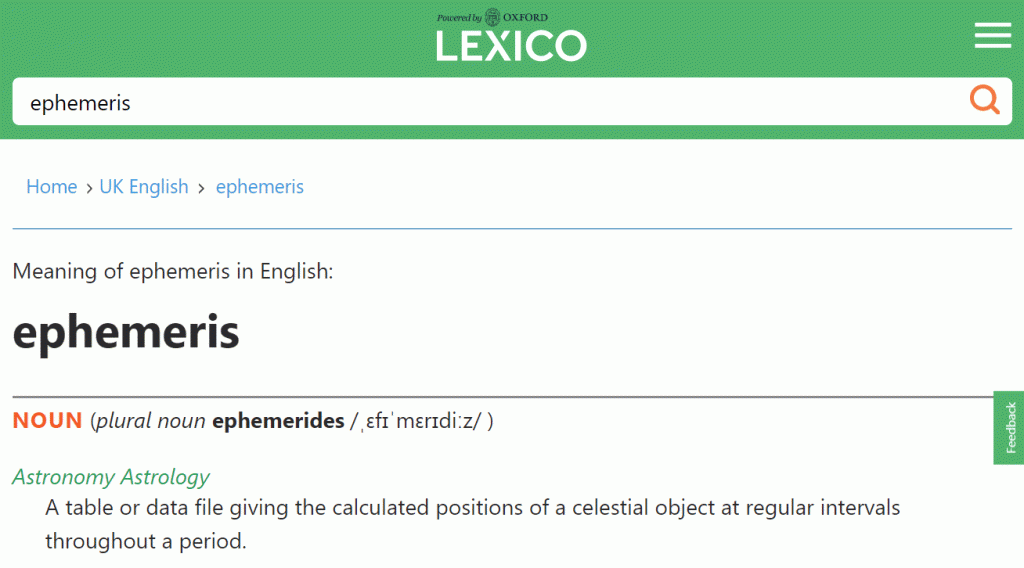
Each satellite also transmits a rough ‘timetable’ for where ALL the satellites in the constellation will be. This is known as the almanac. This helps a GNSS receiver focus on signals from the visible satellites and ignore the unusable ones below the horizon. The almanac data is not essential to provide an accurate fix but it does help speed up the process. It is updated about once a week on the satellites, but an almanac saved on your device will likely be useful for many weeks.
Where is the GPS receiver?
If a GPS receiver knows how far away it is from several known locations then a little geometry allows it to work out where it is. The known locations are the satellites. The term used to describe this simple location method is ‘trilateration’.
Consider a single satellite and a GPS receiver. The receiver already knows it’s on the surface of the earth. It also knows that it must be somewhere on the surface of an invisible sphere, the radius of which is the calculated distance between receiver and satellite.
When two spheres intersect, the periphery of a circle is common to both. The GPS receiver could be anywhere on the red circle in the image below.
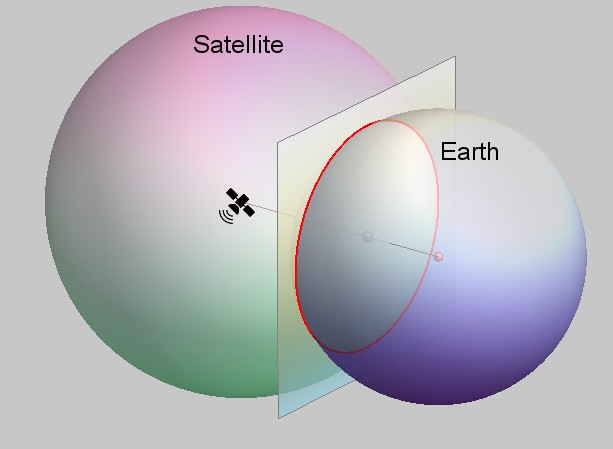
That red circle is shown on the map below. The black drop pin marks the spot where a GPS receiver is. Near Madrid in central Spain. With a signal from just one satellite, the GPS receiver only knows that it’s somewhere on the red circle.
The circle is not to scale but illustrates the point well enough…
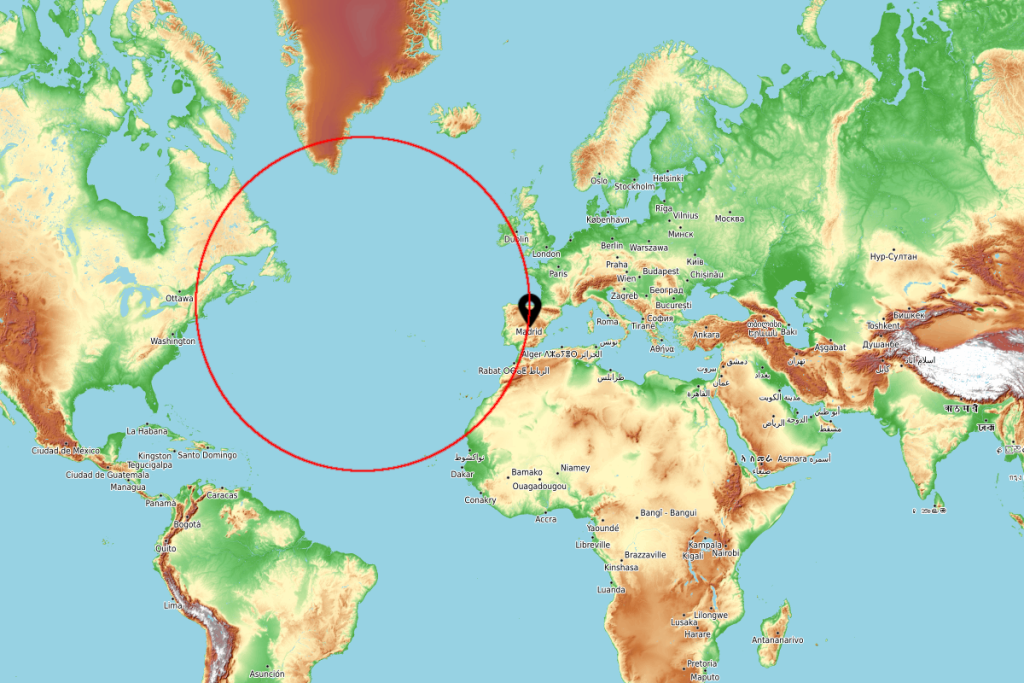
When a second satellite is introduced there are two circles the receiver must be on. There are only two points where the two circles intersect. The GPS now knows it’s near Madrid or just off the SW coast of Iceland but has no way of telling which.
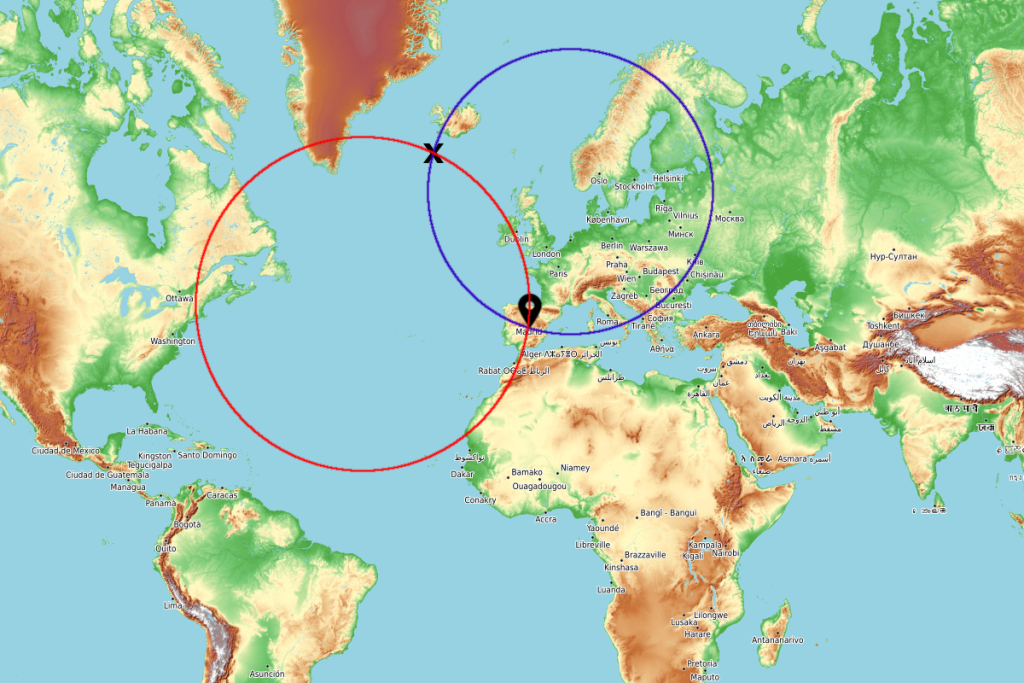
A third satellite is needed. With three satellites there is only one place on earth that’s on all three circles and it’s near Madrid.
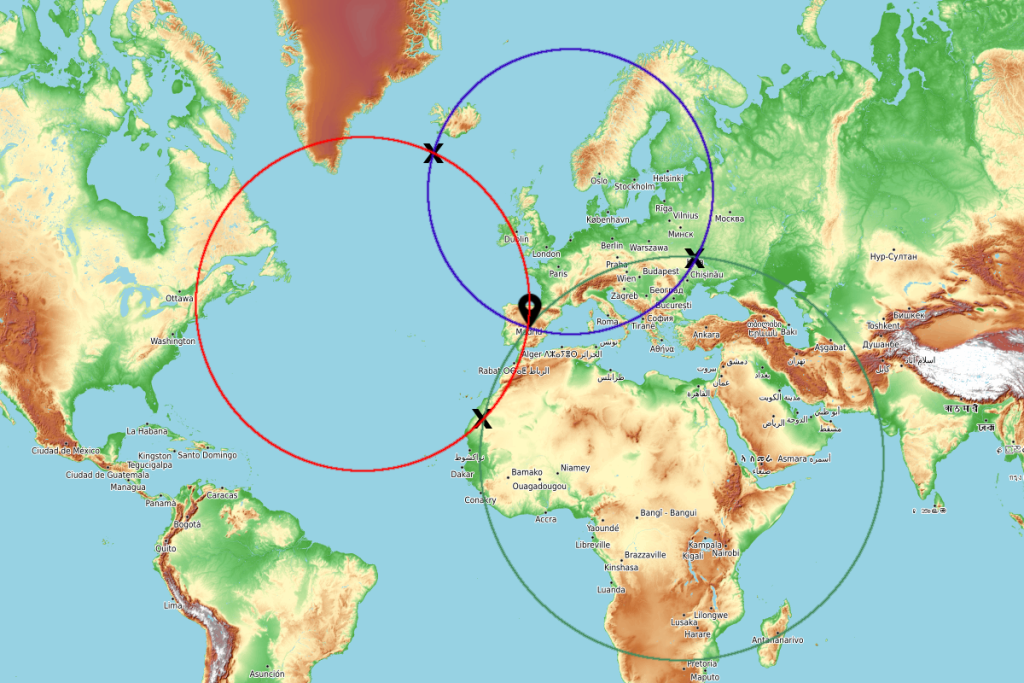
However, the GPS receiver still doesn’t have enough information to know if it’s at sea level or on top of a hill. If you’re on top of a mountain the fix is likely to be particularly poor. Getting a position from only three satellites is called a 2D-fix and it’s unlikely to be very accurate.
Not shown in the 2D maps above, there is a second point that’s common to the intersection of the 3 satellite spheres. That’s well above the earth in space.
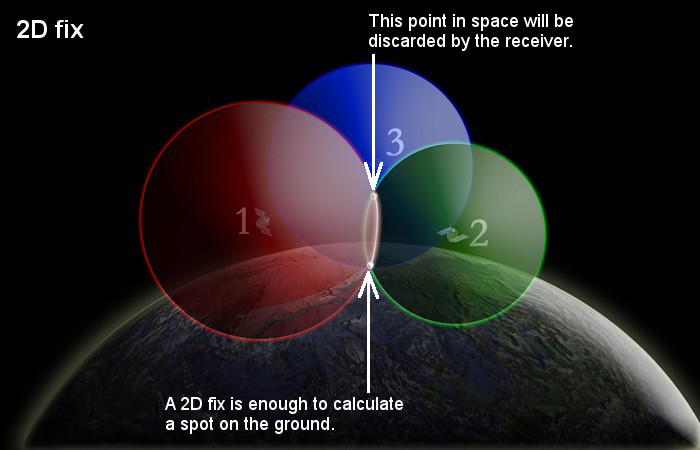
The point in space is discarded for a 2D fix as the GPS receiver knows it’s not in space. A 2D fix must assume the receiver is on the surface of the earth at sea level. To provide an accurate fix that includes elevation, a fourth satellite is needed to define a single point.
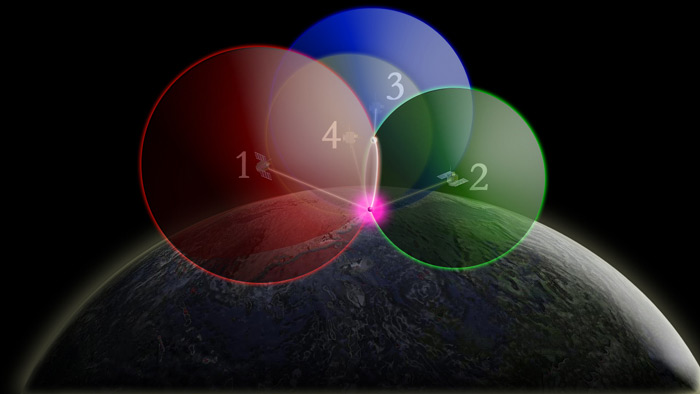
With a fourth satellite a reliably accurate position is provided that shows location and elevation. It also allows the receiver to calculate the exact time from the GPS signals. It no longer has to rely on it’s own relatively inaccurate quartz-based clock. This makes the fix much more accurate. This is known as a 3D-fix.
Four satellites are the minimum for an accurate ‘3D’ fix.
Modern GNSS receivers in phones and GPS handhelds usually process signals from at least two of the global systems and normally many more than four satellites are used to pinpoint position.
This makes getting a fix a quicker, improves the reliability of the accuracy and helps the receiver cope when a proportion of the sky is obscured by trees, cliffs or big buildings etc.
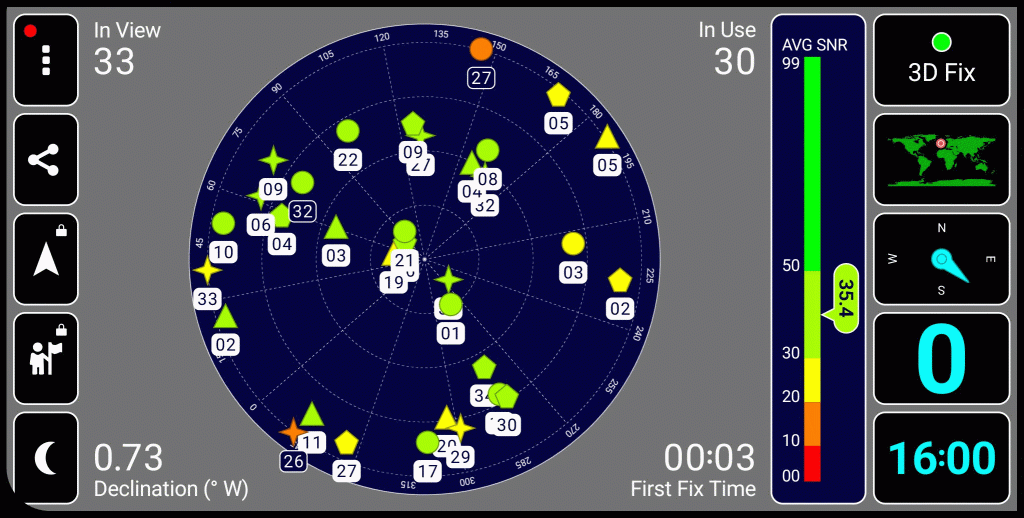
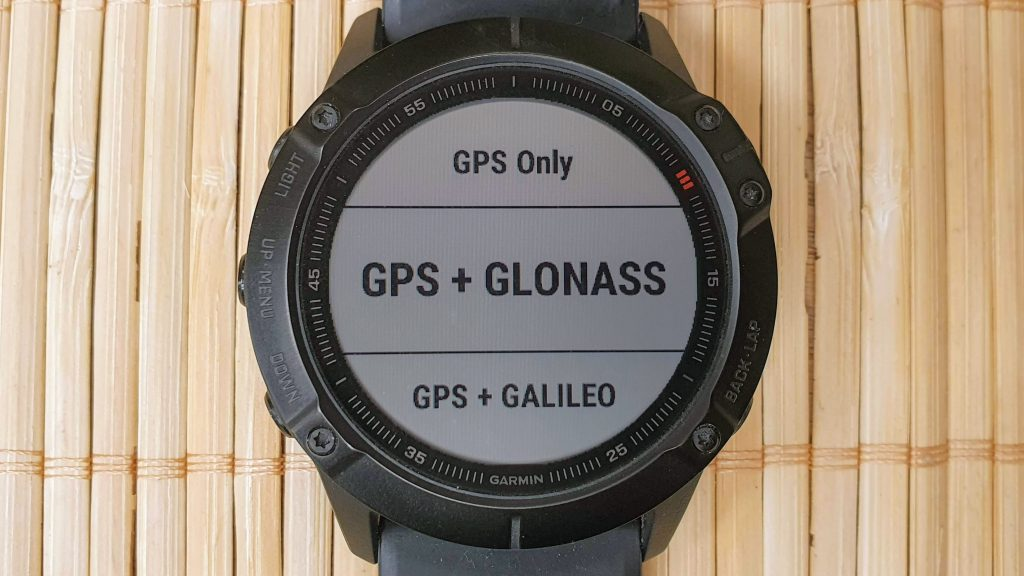
Satellite geometry
Where the satellites are, will affect the accuracy of the calculated fix. Ideally the satellites being used will be well spaced around the sky.
Here’s an example with the minimum of four satellites being used for a fix. When the satellites are well-spaced the point where the four spheres intersect is quite well defined. Errors are likely to be smaller.
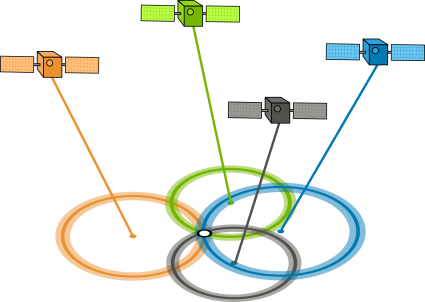
If the satellites are clustered together the fix won’t be so good. The four spheres don’t intersect in a nice clear point.
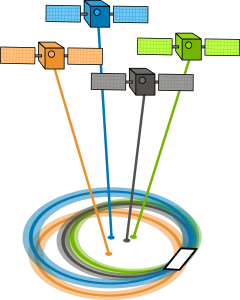
If large sections of the sky are obscured then a good spread of satellites will be hard to achieve. This is one reason why being in rocky gullys or by cliffs or being near taller buildings can spoil the accuracy of your GPS fix.
Also, any satellites that are really near the horizon don’t work so well. The signal will come through more of the ionosphere and troposphere which increases errors.
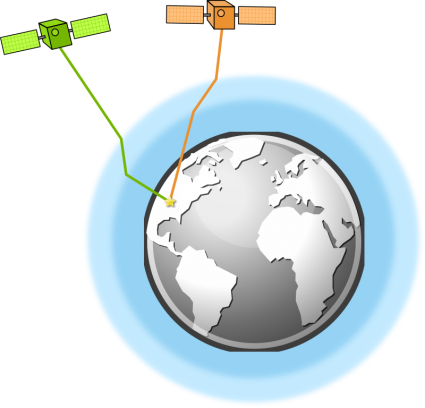
The ideal geometry for four satellites would be to have one directly overhead and the remaining three spaced at 120 degrees around the compass where they are clearly visible, above the horizon.
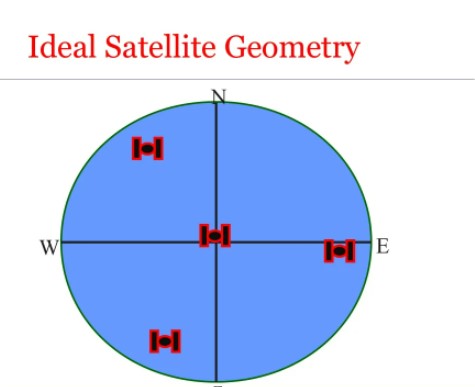
Four satellites enclose an invisible tetrahedron in the sky. The bigger the volume of that tetrahedron, the better the satellite geometry is for providing a reliable fix. That suggests the three spaced satellites should be low in the sky as in the following picture, however if the satellites get too close to the horizon then the signals have to pass through a lot of atmosphere. The geometric gains will eventually be outweighed by increasing atmospheric errors.
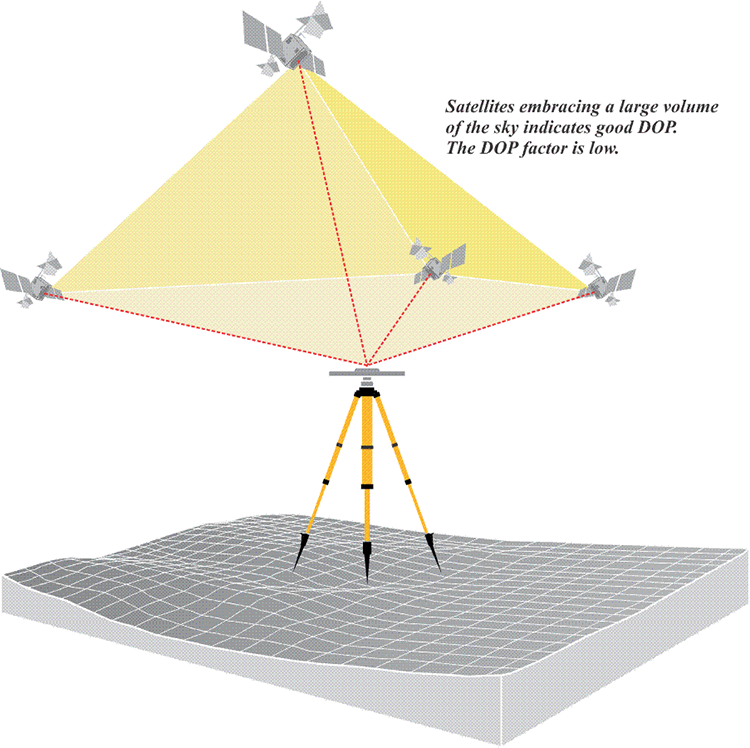
The techie term for the uncertainty relating to satellite geometry is ‘Dilution of Precision’ (DOP).
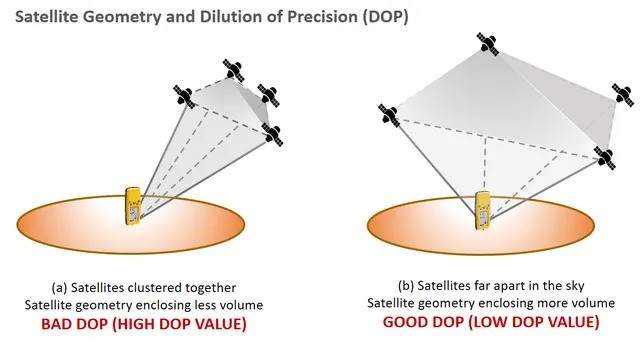
Having some well-spread satellites lower in the sky provides the best geometry for an accurate fix. The next diagram shows a good arrangement for 5 satellites in the lower image.
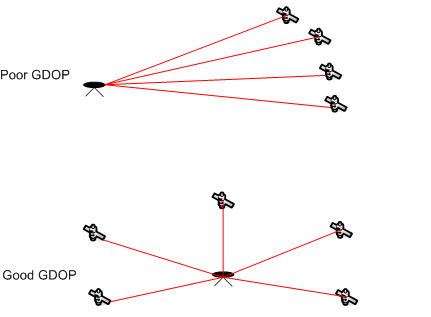
A modern GPS receiver which picks up satellites from multiple GNSS constellations makes this kind of arrangement easy to achieve under an open sky. Here’s a screenshot showing the satellites a Samsung S10e phone was using to provide a 3D fix. With thirty satellites in use, a good 3D fix is no problem.
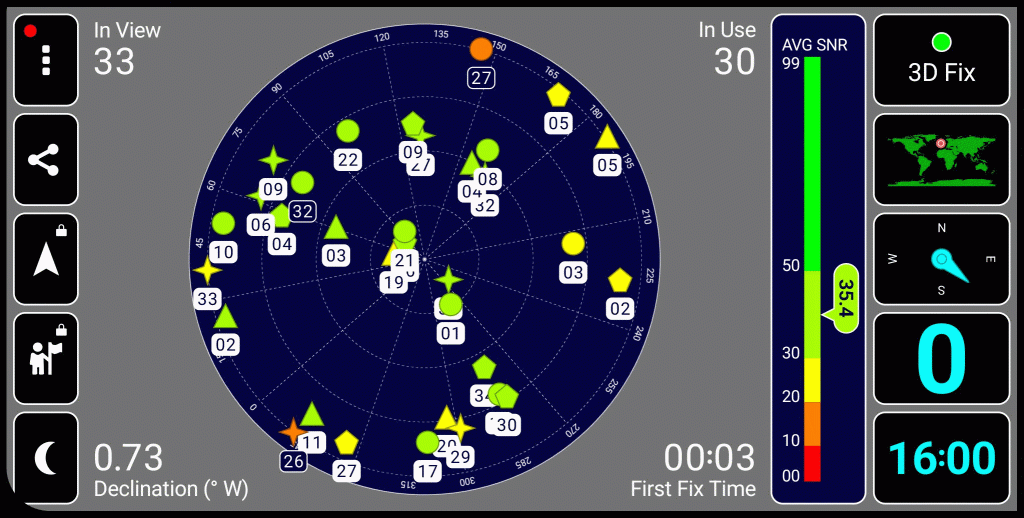
I think that’s probably enough on satellite geometry.
What are we waiting for?
When you switch off a GPS unit it will remember useful information like the almanac, ephemerides, where you were and what the time was. If any of that stored data is still valid next time you use the unit, then it will get it’s first fix more quickly. If the stored data is all wrong then the first fix of the day may take minutes while the receiver gathers up-to-date information.
Hot and cold starts
These terms refer to how quickly the GPS receiver gets a fix when it’s first switched on. A hot start is fast, a cold start, slow. That speed depends on how much valid up-to-date data is still stored on the GPS receiver, remembered from when you last used it.
The following will likely have a slow, ‘cold start’: A brand new GPS handheld, a reset unit, a receiver that hasn’t been used for ages or a device that was last used a long way away (over 100km). A unit needs time to download a valid almanac, ephemeris data, determine the exact time of day and work out where it is. It could take a few minutes.
A ‘warm start’ may occur if you haven’t used the unit for over a day. The accurate ephemeris data may be too old, but the longer lasting almanac will still be valid and the unit should still be telling the right time. If you haven’t moved a long way from where you last used it, you should have your first fix of the day in under 45 secs, probably quicker.
However long that first fix takes, ‘hot starts’ are what you’ll enjoy for the rest of the day. The all-important ephemeris data that describe exactly where the satellites are, will then be up-to-date. Hot starts typically only take a few seconds, 20 secs max.
The data flow from GPS satellites is really slow compared to a home broadband connection. If all the data stored on a GPS unit is out-of-date it may take a few minutes to update itself. If an error occurs whilst downloading data it has to start again, so it doesn’t do any harm to switch a GPS receiver on when you first arrive at the start of your adventure. If you give it time to get it’s first fix then it’ll be ready to go when you set off.
A smartphone uses it’s built-in GNSS receiver for so many things that it may already have up-to-date data. Smartphones can also download almanac and ephemeris data over an internet connection, using A-GPS (Assisted GPS). That’s a trick most handhelds can’t perform. So don’t be surprised if your phone beats a fancy handheld to the first fix of the day. However, A-GPS doesn’t make a smartphone any more accurate, just quicker to download the data.
For a minute or so after any fix has been provided the given position will get reliably more accurate as more satellites are picked up. They improve ‘confidence’ in the exact position.
If your device always takes a long time to get a fix then there’s probably something wrong (over 30 seconds). A failing connection between the aerial and the receiver chipset is a potential culprit.
If the battery life on your preferred GPS device is plenty good enough for your planned outing, you can just leave the unit running all the time. Then you won’t have to wait at all for a fix. A glance at the map on the GPS unit will instantly reveal where you are.
The Garmin ‘Enduro 2’ GNSS watch can go non-stop for nearly 110 hours. Garmin handhelds usually run non-stop for at least 16 hours, the GPSMAP67 may manage 180 hours. Many smartphones will not last nearly so well and it’s best to use phones sparingly.
What’s the time?
Knowing the right time is essential for a GNSS to work. The speed of light is about 300,000 km per second and it only takes 1/15th of a second for a signal to reach earth from a satellite that’s directly overhead. A timing error of a millionth of a second is enough to introduce a 300 metre location error.
Each of the satellites are equipped with multiple atomic clocks to help keep super-accurate time. These are the most accurate timing devices we’ve ever invented. The ones used on GPS satellites keep time to billionths of a second. (timeandnavigation.si.edu).
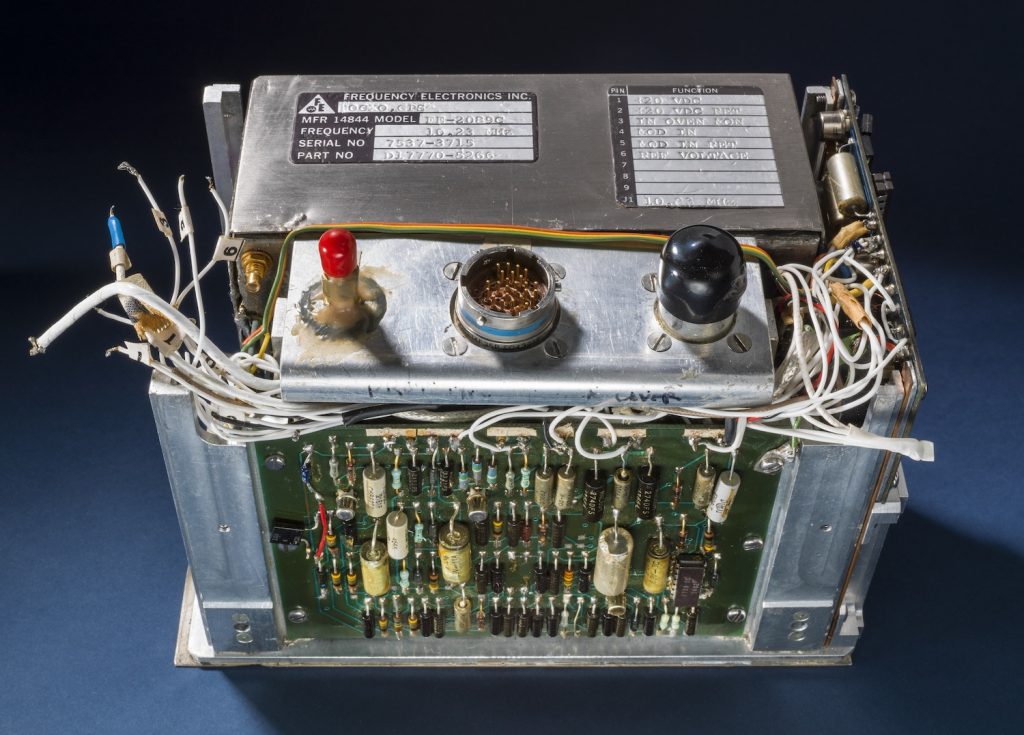
The atomic clocks installed in satellites have to be light and able to withstand the rigours of space. Even though they are incredibly accurate, their natural drift is still corrected by even more stable, fridge-sized atomic clocks on earth, twice a day (NASA.gov).
You might be wondering how any of this works when our smartphones and handhelds only have relatively inaccurate quartz crystal clocks in them. In fact, a GNSS receiver can eliminate any internal clock error using the time signals from the satellites.
It’s true that a quartz-based clock in a consumer device does not keep time well enough for GPS-accurate distance calculations. It’s clock will be ‘out’ but the unknown error will be identical for all the received signals. You might remember from solving equations at school that you need two equations to solve for two unknowns. The same is true for GPS except there are four unknowns and four equations to solve. The unknowns are the three dimensions that define exactly where the GPS receiver is and time.
Once the receiver is successfully receiving timing signals from four satellites it has enough information to solve those four equations. It works out exactly what your receiver clock error is and uses the corrected time for all the positioning calculations. Clever stuff. GPS effectively turns your smartphone or GNSS unit into a very accurate clock indeed.
Four satellites will provide accurate location, elevation and the exact time.
It’s all relative
So critical is the accuracy of the timing signals that relativistic effects must be taken into account. We know from Einstein that time flows at a different rate on the satellites than it does on earth. They are moving at nearly 8,700 mph. This has the effect of slowing the satellite clocks by 7.2 millionths of a second a day (7.2 μs/day) compared to the clocks on earth.
Additionally, the effects of gravity are less than on earth because the satellite orbits are so high. This has the effect of speeding up the clocks by 45.8 μs/day. The net effect is that the clocks on GPS satellites run faster than clocks on earth by (45.8 – 7.2) = 38.6 μs every day.
GPS only works if the clocks on the satellites are perfectly synchronised with each other, and with the time on earth. So, the satellite clocks are made to run more slowly by 38.6 μs/day. Wow.
Theory | Value | Notes |
---|---|---|
SR (Special Relativity) | -7.2 μs/day | Clocks run slower in satellites due to velocity |
GR (General Relativity) | +45.8 μs/day | Clocks run faster in satellites due to lower gravity |
Total (Combined) | +38.6 μs/day | GR is larger effect than SR |
If these timing corrections weren’t made then any GNSS would quickly become useless. After just 24 hours, location errors would be nearly 12 kilometres.
300,000 x (38.6 ÷ 1,000,000) = 11.58 km
If you want to know the right time, check your GPS device 🙂